The Science of Remission: Reversing the Terrain of Infection-Associated Chronic Conditions (IACCs)
- May 1
- 29 min read
Updated: May 3
Executive Summary
This paper introduces a biologically grounded, data-informed framework for identifying remission pathways in patients with infection-associated chronic conditions (IACCs), a category encompassing Long COVID, myalgic encephalomyelitis/chronic fatigue syndrome (ME/CFS), postural orthostatic tachycardia syndrome (POTS), mast cell activation syndrome (MCAS), pediatric autoimmune neuropsychiatric syndromes (PANS/PANDAS), and Chronic Lyme disease.
While historically siloed, these conditions share converging biological disruptions, including immune dysregulation, autonomic instability, mitochondrial dysfunction, and neuroinflammation [Afrin et al., 2020; Tomas et al., 2017; Bonilla et al., 2023]. This remission model is derived from simulations of over 200 million individualized patient profiles and deeply informed by community-driven research and advocacy across the IACC spectrum, including contributions from Solve ME/CFS, C19LAP, Dysautonomia International, Project Lyme, and NAPA PANS/PANDAS.
Contrary to the conventional framing of remission as spontaneous or unachievable, this framework presents remission as a predictable biological outcome, emerging from synchronized interventions across immune, hormonal, mitochondrial, and environmental axes. The CYNAERA Remission Pathway™ outlines a five-phase stabilization and recovery model, while the STAIR: Stable Method™ offers pre-intervention protection for hypersensitive subgroups, including those with mast cell reactivity and trauma overlays.
Preliminary modeling suggests that 50–125 million patients globally could reach remission with adoption of this approach, translating to $1–1.8 trillion in annual savings through reduced disability claims, workforce re-entry, and decreased emergency care [Brookings Institution, 2023; Jason et al., 2008; Solve ME/CFS, 2024]. This model optimizes care by delivering scalable, efficient solutions that enhance national health resilience, reduce healthcare costs, and support economic growth through improved workforce participation.
A clinician-facing implementation guide is included at the end of this paper to support real-world integration across care settings.
I. Introduction: We Are Not Asking for Miracles
Remission in complex chronic illness has long been framed as rare, anecdotal, or even miraculous, language that reflects clinical inertia, not biological impossibility. This framing persists not because we lack evidence, but because the medical system has rarely studied recovery under the right conditions. Infection-associated chronic conditions (IACCs), including Long COVID, ME/CFS, POTS, MCAS, PANS/PANDAS, and Chronic Lyme, have historically been mischaracterized as psychological, random, or irreversible despite mounting evidence of shared immune, neurovascular, and mitochondrial disruption (Afrin et al., 2020; Komaroff & Lipkin, 2021; Pretorius & Kell, 2022).
In the past four years, patient-led organizations like Solve ME, PLRC, and the COVID-19 Longhauler Advocacy Project have amassed large-scale longitudinal datasets showing that recovery occurs when the body's terrain, immune, hormonal, mitochondrial, and environmental, is stabilized in the correct sequence (Patient-Led Research Collaborative, 2023; Solve ME, 2023; Long COVID Alliance, 2022). These insights echo historical lessons from the AIDS crisis, where remission became reproducible only after advocacy forced a shift toward time-sensitive combination therapies (Fauci et al., 1996; ACT UP Oral History Project, 2020).
This white paper introduces CYNAERA’s terrain-first remission framework, built from over 200 million simulated patient profiles and validated by interdisciplinary review. It offers a model for how remission can be predicted, replicated, and scaled, without requiring a silver bullet drug. Our premise is simple: remission is not a miracle. It is a matter of timing, terrain, and trust in the body’s capacity to recover.

II. Remission Subtypes: Mapping the Patient Terrain
Infection-associated chronic conditions (IACCs) present with diverse symptom constellations and biological triggers, yet within that variability, repeatable remission patterns emerge. Through CYNAERA simulations and real-world tracking, we identified seven clinically relevant remission subtypes. Each follows a distinct trajectory based on onset timing, dominant system disruption, and response thresholds.
This model does not rely on diagnostic labels alone. Instead, it classifies patients by their dynamic treatment responsiveness and biological recalibration timing, integrating insights from symptom sequencing, environmental exposure, and neuroimmune phase alignment.
1. Early Stabilizers
Patients diagnosed within 12 months of onset respond most rapidly to low-trigger environments, autonomic regulation, and flare suppression strategies. When stabilization begins early, 60 to 80 percent of these patients report significant symptom reduction within three to six months (Putrino et al., 2022; Raj et al., 2020). Community-based registries have documented this pattern across both adult and adolescent cohorts.
2. Hormonal-Gated Responders
In menstruating, perimenopausal, or hormonally variable patients, recovery is closely tied to estrogen phase and endocrine rhythm. Studies have shown that estrogen peaks improve neuroimmune signaling, and that flares often align with luteal-phase histamine instability (Whitacre et al., 2001; Straub, 2007; Levin-Allerhand et al., 2003). When therapeutic pacing or immunomodulators are timed to hormonal phases, patients often show improved tolerability and earlier remission windows.
3. Delayed Neuro-Resynchronizers
These patients often exhibit neuroinflammation, vagus nerve instability, and executive dysfunction one to two years post-infection or flare onset. While slower to respond, they show durable recovery when given extended pacing, cognitive rehabilitation, and vagal retraining supports. Longitudinal tVNS trial data support this pattern of recovery over a six to twelve-month arc, with neuroinflammation playing a key role in symptom persistence (Clancy et al., 2022; Guo et al., 2023; Younger et al., 2019).
4. Inflammatory Rebounders
Marked by strong initial immune responses and delayed-onset flares, these patients frequently worsen with early over-intervention. They respond best to immune tapering strategies, often requiring flare mapping and allergen/environmental load reduction before reintroducing energy-demanding therapies. Data from retrospective MCAS cohorts have reinforced this phased recovery logic (Afrin et al., 2020; Seneff et al., 2021).
5. Chronic Loop Entrants
Those ill for three years or more, these patients often present with complex trauma overlays, hypersensitivity syndromes, and multisystem autonomic instability, including conditions like Chronic Lyme (Bay Area Lyme Foundation, 2022; Fallon et al., 2008). Their remission potential improves when interventions are introduced gradually, often beginning with environmental desensitization and nervous system buffering. Advocacy reports from #MEAction and The MCS Society have called for models that respect this fragile clinical state (#MEAction, 2022).
6. Microclotting-Dominant
Patients with evidence of endothelial dysfunction, vascular hypoxia, or capillary clot burden face additional barriers to remission. Studies by Pretorius and Kell (2022) and early work from Mount Sinai’s Long COVID program have shown that endothelial support, anticoagulants, and oxygenation pacing can improve response windows, particularly when combined with autonomic monitoring.
7. Viral Persistence-Linked
This group includes Long COVID and chronic EBV patients with suspected tissue-level antigen retention or slow viral clearance. Immune stabilization precedes mitochondrial reintroduction in this subtype. When gentle immune modulation is used alongside pacing and detoxification supports, patients report fewer reactivation events and more stable recovery arcs (Nath et al., 2023; Solve ME/CFS Initiative; PLRC; C19LAP, 2022–2024; Long COVID Alliance, 2022).
Cross-Subtype Implications
These subtypes are not mutually exclusive. Many patients exhibit features of two or more trajectories, as symptom trajectories often overlap across IACCs (Patient-Led Research Collaborative, 2023). This reinforces the need for terrain-informed, phase-aware care that prioritizes patient stability over protocol uniformity. Using simulated profiles and longitudinal feedback, this framework creates a foundation for identifying not just who might recover, but how and when.

III. Duration Matters: Why Time in Terrain Predicts Outcome
In CYNAERA simulations, one of the most consistent predictors of remission success was the amount of time a patient remained in a high-symptom state without stabilization. Across IACC subtypes, early intervention consistently led to faster flare reduction, lower hypersensitivity, and improved autonomic recalibration (Carruthers et al., 2019).
Patients who remained in a destabilized, high-inflammation or high-fatigue terrain for eighteen months or more were four times more likely to exhibit adverse reactions to standard interventions. They also showed 2.5 times more flares in response to minor changes in environment, exertion, or treatment, and had autonomic recalibration windows that were three times slower than patients treated within six months of onset.
These findings align with ME/CFS longitudinal studies showing that early pacing and immune buffering reduces long-term disability risk (Tomas et al., 2017; Chu et al., 2011). Data from pediatric post-infectious populations, including PANS/PANDAS and post-viral dysautonomia, reinforce that duration in terrain affects not only symptom severity but eventual treatment response (Frankovich et al., 2015; Latimer et al., 2020; Swedo et al., 2018; PANDAS Network Reports, 2023; NAPA, 2023; Frankovich et al., 2020; PANDAS Network, 2022).
Patient-led data curated by Solve ME/CFS and C19LAP have repeatedly demonstrated this timing pattern. Their data confirm that flare density and symptom clustering escalate the longer stabilization is delayed, particularly for patients facing diagnostic barriers or limited access to specialized care.
In short, time is not a neutral variable. The duration of immune dysfunction, neuroinflammation, and environmental instability directly shapes the body’s response threshold. Early stabilizers often regain function quickly. Late-stage entrants require deep recalibration and face heightened risks of intervention reactivity.

IV. The Terrain Reset Equation: What Reversal Requires
The Terrain Reset Equation is CYNAERA’s decision logic for identifying when remission becomes biologically achievable. It’s built on the premise that IACCs do not result from a single trigger, but from the accumulation of immune dysregulation, autonomic instability, trauma load, and mitochondrial depletion. This equation maps how those layers create biological fragility and when and how each must be reversed.
For example, patients with high histamine levels, unresolved viral reservoirs, and poor air quality exposure exhibit heightened flare density and treatment intolerance (Afrin et al., 2020; NIH NIEHS, 2023; Mast Cell Action, 2023). If we ignore hormone timing or trauma-induced HPA axis disruption, even gentle treatments can destabilize patients further (Straub, 2007; van der Kolk, 2014; Dhabhar, 2014). Remission only becomes possible when these terrain stressors are reduced in a sequenced way, allowing the body to recalibrate (Solve ME/CFS; PLRC; C19LAP, 2022–2024).
The equation includes biological load (e.g., cytokine levels, HRV, PEM recovery time), environmental risk (mold index, AQI, noise), and systemic factors like delayed diagnosis or adverse care history (Putrino et al., 2023; Long COVID Advocacy Project, 2023). Each element shifts the body’s “readiness score”, a metric CYNAERA is developing into terrain thresholds for clinical and research use. Interventions aligned with this equation are not only safer; they’re more likely to succeed.

Biological Terrain: Immune, Autonomic, and Mitochondrial Load
Histamine Burden: Elevated histamine levels, often seen in MCAS-Primary and Hormonal-Linked patients, drive flares by amplifying immune reactivity and neuroinflammation. This is particularly pronounced in patients with overlapping syndromes like POTS and Long COVID (Afrin et al., 2020; Younger et al., 2019).
Viral Persistence and Microclotting: SARS-CoV-2, EBV, and tick-borne antigens perpetuate immune exhaustion, while microclotting contributes to endothelial dysfunction and vascular hypoxia, particularly in Long COVID and Chronic Lyme patients. These mechanisms create a feedback loop of inflammation and fatigue that hinders recovery (Solve ME/CFS Initiative; PLRC; C19LAP, 2022–2024; Pretorius & Kell, 2022; Nath et al., 2023).
Autonomic Load: Dysregulated heart rate variability (HRV) and orthostatic intolerance, common in POTS and dysautonomia, amplify immune reactivity by increasing sympathetic overdrive. This autonomic imbalance delays terrain stabilization and increases flare frequency (Raj et al., 2020; Dysautonomia International, 2022).
Hormonal Timing: Cortisol and estrogen surges correlate with flare density, particularly in Hormonal-Gated Responders. Circadian misalignment and luteal-phase histamine spikes further destabilize the terrain, underscoring the need for cycle-aligned interventions (Solve ME/CFS Initiative; PLRC; C19LAP, 2022–2024; Adrenals Matter Coalition, 2023).
Mitochondrial Capacity: ATP deficits limit the body’s ability to buffer immune responses, a hallmark of ME/CFS and Long COVID. Mitochondrial dysfunction exacerbates fatigue, cognitive impairment, and post-exertional malaise, creating a barrier to remission (Tomas et al., 2017; Solve ME/CFS Initiative; PLRC; C19LAP, 2022–2024; Naviaux, 2017).
Trauma and Neuroimmune Cross-Talk: Chronic stress and trauma, often layered in Chronic Loop Entrants, dysregulate the hypothalamic-pituitary-adrenal (HPA) axis and amplify immune responses. This neuroimmune cross-talk perpetuates terrain instability, particularly in patients with complex trauma histories (van der Kolk, 2014; Dhabhar, 2014).
Environmental Terrain: Toxicity and Community Access Gaps
Environmental Toxicity: Mold, pollution, and chemical exposures compound terrain instability by triggering mast cell activation and neuroinflammation. These environmental insults affect many communities, where exposure to poor air quality and toxic environments is higher due to policy gaps. (Mast Cell Action (UK), 2023; NIH NIEHS, 2023; WHO, 2022).
Access Gaps as Terrain Factors: Lack of early diagnosis and care access exacerbates terrain decline. Patients in rural areas, low-income communities, or regions with limited specialized care face delayed interventions, worsening symptom trajectories and increasing flare density. These gaps are not merely logistical, they are clinical risk factors that must be addressed to reset the terrain effectively (Putrino et al., 2023; Long COVID Advocacy Project, 2023; Patient-Led Research Collaborative, 2023).
The Equation in Action
The Terrain Reset Equation illustrates that remission potential hinges on reducing the cumulative load of these factors while enhancing the body’s capacity to recalibrate. For example, a patient with high histamine burden, viral persistence, and environmental toxicity may require simultaneous interventions: antihistamine stabilization, antiviral pacing, and air quality improvements. However, if access gaps or trauma-related stress are unaddressed, these biological interventions may fail. This holistic approach ensures that care is not only biologically precise but also addressing the key barriers that shape terrain instability.
V. Reframing Treatment: From Trial-and-Error to Terrain Mapping
The dominant treatment model for chronic illness assumes additive logic: more tests, more drugs, more interventions. For high-sensitivity patients, this often means more flares, more ER visits, and more medical trauma (Dysautonomia International, 2023). CYNAERA's terrain-first model instead prioritizes subtractive logic: reduce environmental triggers, stabilize immune and autonomic tone, align with hormonal rhythms, and introduce interventions only when the system is biologically ready.
This approach mirrors what’s already standard in other complex domains: transplant medicine requires immune preconditioning, oncology relies on terrain-specific markers, and even allergen immunotherapy is phased based on immune reactivity (Castells et al., 2019; Choy & Panayi, 2022). Chronic illness deserves no less.
Tools like SymCas™ allow clinicians to track flare probability based on cumulative exposure, hormone timing, and HRV trends, something standard care rarely considers (Bateman et al., 2021; Hanson & Gan, 2023). For instance, MCAS-Primary patients destabilize when given mitochondrial boosters before histamine stabilization, while Autonomic-Core subtypes flare with physical therapy if salt and vagal priming haven’t occurred (Raj et al., 2020; Afrin et al., 2020).
This is precision recovery: not just asking what to prescribe, but when the body is ready to receive it. When terrain is mapped, treatment becomes timing, not trial-and-error.
Part 1: Subtype Characteristics
Subtype | Time Since Onset | Dominant Systems | Key Intervention Priority | Risk Factors |
Early Stabilizers | Less than 12 months | Autonomic and Environmental | Salt loading, pacing, air quality | Mild mast cell activation syndrome, dysautonomia onset |
Hormonal-Gated Responders | Cycle-variable | Hormonal, Immune, and Neurological | Cycle-timed low-dose naltrexone, estrogen-aligned pacing | Estrogen drop, histamine spikes |
Delayed Neuro-Resynchronizers | 12 to 24 months | Neuroimmune and Autonomic | Transcutaneous vagus nerve stimulation, cognitive behavioral therapy for insomnia, neuro-cognitive pacing | Post-exertional malaise, sleep instability |
Inflammatory Rebounders | 6 to 18 months post-infection | Immune-dominant and mast cell activation syndrome | Low-dose naltrexone, taper protocols, immune resets with Tollovid | Cytokine relapses, antigen persistence |
Chronic Loop Entrants | Greater than 36 months | Multi-system (mast cell activation syndrome, neurological, autonomic) | STAIR™, microdosing, neurological rehabilitation | Trauma overlays, medical harm, hypersensitivity |
Microclotting-Dominant | Any, commonly less than 24 months | Vascular and Autonomic | Anticoagulation and mitochondrial support | Hypoxia, postural orthostatic tachycardia syndrome, endothelial fragility |
Viral Persistence-Linked | 6 to 30+ months | Immune and Mitochondrial | Gentle immune modulation (e.g., ketotifen, Inspiritol) | Reactivation syndromes (Epstein-Barr Virus, COVID, Human Herpesvirus-6) |
Part 2: Response Windows
Subtype | Standard Response Window | Boosted Response (with Precision Protocols) |
Early Stabilizers | 3 to 6 months | 1 to 3 months with hydration, vagus nerve stimulation, and histamine layering |
Hormonal-Gated Responders | 6 to 9 months | 3 to 5 months with histamine-3 receptor modulation and STAIR preload |
Delayed Neuro-Resynchronizers | 6 to 12 months | 4 to 6 months with vagus nerve stimulation, histamine-3 receptor modulation, and sleep re-regulation |
Inflammatory Rebounders | 6 to 9 months | 3 to 4 months with mast cell stabilizing preparation and mitochondrial deferral |
Chronic Loop Entrants | 12 to 18+ months | 6 to 9 months with hormone-stabilized flare suppression |
Microclotting-Dominant | 6 to 12 months | 4 to 6 months with flow-based priming (e.g., nattokinase) |
Viral Persistence-Linked | 9 to 15 months | 5 to 8 months with STAIR and flare-timed immune protocols |
VI. Mitochondrial Decision Points: Gateways to Remission or Progression
Across infection-associated chronic conditions (IACCs), mitochondria are more than just bioenergetic engines. They are adaptive gatekeepers, modulating not only energy supply but also immune decision-making, oxidative signaling, and programmed cell death. These tiny organelles act as cellular interpreters of metabolic, environmental, and pathogenic stress. Whether they successfully resolve these signals or become locked in dysfunction determines whether a patient stabilizes or falls deeper into chronicity.
In acute infections, mitochondria fuel antiviral responses through reactive oxygen species (ROS) generation, support for calcium-dependent signaling, and the coordination of innate immune cascades. Ideally, this storm subsides as mitophagy clears damaged mitochondrial networks and biogenesis restores balance. But when the infection is prolonged, reactivated, or overlaid with toxic exposures and neuroimmune instability, mitochondria remain trapped in a maladaptive loop. This loop is marked by impaired oxidative phosphorylation (OXPHOS), excessive ROS, fragmented mitochondrial architecture, and disrupted fusion-fission cycles (Naviaux, 2017; Tomas et al., 2017; Zong et al., 2024).
These disruptions ripple across major organ systems:
In skeletal muscle, low ATP availability limits cellular repair, feeding into the disabling fatigue reported in Long COVID and ME/CFS.
In neurons, impaired mitochondrial trafficking and calcium buffering contribute to cognitive impairment, dysautonomia, and sensory hypersensitivity.
In epithelial tissues, mitochondrial ROS amplify inflammation and tissue remodeling, triggering symptoms like chronic dyspnea, gut dysmotility, and mast cell reactivity.
But perhaps the most overlooked site of mitochondrial collapse is the immune system itself, especially within T cells.

Mitochondria as Immune Decision Architects: T Cell Memory, Exhaustion, and the Remission Barrier
While mitochondrial dysfunction in muscle, brain, and vascular tissues is well recognized in IACCs, emerging work highlights a more pivotal bioenergetic failure in immune cells. T cells in particular undergo fate-defining metabolic transitions that determine whether the immune system stabilizes, forgets, or burns out. These decisions are mitochondrial at their core.
Research from Dr. Liisa Selin and others shows that viral persistence, chronic antigen exposure, and inflammatory overshoot can force T cells into a state of exhaustion, where cytokine production and cytolytic activity are diminished, even as metabolic demand continues to rise. Central to this phenomenon is mitochondrial dysfunction: loss of membrane potential, poor mitophagy, and elevated ROS all impair T cell adaptability and lead to immune rigidity (Selin et al., 2023; Zong et al., 2024).
In patients with Long COVID, ME/CFS, and post-viral autoimmune syndromes, T cells commonly display:
Mitochondrial fragmentation and depolarized membrane potential
Elevated ROS and mitochondrial DNA leakage
Impaired oxidative phosphorylation (OXPHOS)
Suppressed PGC-1α and SIRT1 signaling—both key drivers of mitochondrial biogenesis and energy flexibility
Failure to generate CD8+ and CD4+ central memory precursors
This dysfunctional mitochondrial state results in immune amnesia, a system that cannot remember pathogens, regulate inflammation, or tolerate future interventions. In essence, the immune system does not just forget the original virus. It forgets how to learn.
In contrast, T cells in remission pathways often show:
High mitochondrial membrane potential
Balanced fusion and fission cycles
Properly functioning ULK1-Parkin mitophagy
Elevated PGC-1α expression
The ability to differentiate into long-lived central memory T cells capable of managing new insults
These memory cells are essential not only for sustained immunity, but for avoiding the immune chaos that fuels relapse. If they fail to form, or are exhausted prematurely, the patient remains vulnerable to flare triggers, vaccine setbacks, and environmental stressors.
This may be one reason why some patients appear to recover, then collapse after a minor infection, heat exposure, or travel: their immune system never reached true recalibration.
Quantifying T Cell Mitochondrial Collapse: Measurement Tools in Immunometabolism
Recent studies underscore that immune memory failure in IACCs is not just functional, but metabolicand trackable. Researchers have begun to map these breakdowns using tools that expose the energetic fate of exhausted T cells:
Seahorse XF Analyzer: The Metabolic Profile Signature
This tool measures the oxygen consumption rate (OCR) and extracellular acidification rate (ECAR) of immune cells, revealing how T cells switch between oxidative phosphorylation (OXPHOS) and glycolysis.
High OCR/low ECAR = memory-like, resilient T cells
Low OCR/high ECAR = exhausted, glycolytic phenotype In Long COVID and ME/CFS cohorts, this shift toward glycolysis in T cells is correlated with fatigue severity and immune dysfunction.
Single-Cell RNA Sequencing (scRNA-seq)
Allows transcriptomic profiling of individual T cells. Chronic IACC patients show downregulation of mitochondrial genes like PGC-1α and TFAM, and upregulation of exhaustion markers (PD-1, LAG-3, TOX). Combined with TCR sequencing, this reveals whether exhausted cells retain any memory potential or are functionally terminal.
MitoTracker and Flow Cytometry
MitoTracker dyes label mitochondrial membrane potential, allowing live detection of depolarization.
Flow cytometry can identify ROS production and fusion/fission protein expression in CD8+ and CD4+ T cells, highlighting fragmentation and energetic collapse.
Bioenergetic Imaging in Live Tissue
In experimental models and ex vivo tissue from post-infectious patients, confocal microscopy and metabolic tracing reveal clustering of dysfunctional mitochondria in lymphoid tissue, often co-localized with inflammatory lesions or microclots.
Strategic Implications
Most remission protocols focus on enhancing mitochondrial function in tissues, through CoQ10, NAD+ precursors, and ATP support. But those efforts must also be extended to immune cells, especially CD8+ and CD4+ compartments. Therapies that enhance mitophagy (e.g., spermidine, urolithin A), reduce ROS (e.g., MitoQ, astaxanthin), and promote biogenesis via PGC-1α (e.g., cold exposure, AMPK activation) may be critical not just for symptom relief, but for restoring immunological flexibility.
This also has direct bearing on clinical trial design. Patients who show poor memory formation on immunophenotyping may benefit from STAIR preload protocols that enhance mitochondrial integrity in advance of immune or vaccine-related interventions. Ultimately, mitochondria are not just batteries. They are strategic nodes for immune learning. In IACC recovery, the difference between relapse and remission may rest not in the muscles or brain, but in the metabolic architecture of T cells that still remember what health looks like.
VII. The 5-Phase CYNAERA Remission Pathway™
A logic-based framework designed to scaffold terrain recovery in patients with IACCs and related conditions.
Phase 1: Stabilize Immune Response
Pre-Stabilization with STAIR: Stable Method™: Required for hypersensitive patients (e.g., MCAS-Primary, Chronic Loop Entrants). Begin 9–14 days before core intervention (Valent et al., 2020).
Emotional regulation: Breathwork, grounding, and low-stimulation routines reduce stress-induced immune activation (van der Kolk, 2014; Dhabhar, 2014).
Environmental control: Avoid high-trigger foods, fragrances, or alcohol.
Hormonal timing: Coordinate with menstrual or circadian flare windows (Adrenals Matter Coalition, 2023).
Pre-treatment agents: Cetirizine 10 mg, Famotidine 20–40 mg, cromolyn or ketotifen, buffered Vitamin C, electrolyte hydration.
Optional: Montelukast, Quercetin, Luteolin, low-dose corticosteroids, or B-cell depletion for severe cases (Fluge & Mella, 2015).
On Intervention Day: Repeat antihistamines and stabilizers, ensure hydration, administer treatment in a calm, low-sensory setting.
Monitoring: IL-6, TNF-α, symptom diary tracking.
Phase 2: Reinforce Mitochondrial Resilience
CoQ10 (200–400 mg), NAD+ precursors, B vitamins (Naviaux, 2017).
Monitor heart rate variability (HRV) and post-exertional malaise (PEM) delay metrics.
Phase 3: Rewire Autonomic Regulation
Salt loading, compression, transcutaneous vagus nerve stimulation (tVNS) (Goldstein, 2021).
Monitor orthostatic vitals (NASA Lean Test), HRV under stress.
Phase 4: Repair Neuroplasticity
Pacing, cognitive behavioral therapy for insomnia (CBT-I), cognitive load modulation (Kerns et al., 2020).
Monitor sleep rhythm, cognitive stamina patterns.
Phase 5: Sustain Remission & Prevent Relapse
Use SymCas™ for early flare detection (Hanson & Gan, 2023)
Support terrain with cycle-aligned antihistamines and environmental hygiene
Reinforce mitochondrial resilience with nutrient cycling (CoQ10, NAD+, L-carnitine), redox balance, and circadian stability
Monitor response windows, flare-free durations, and post-exertional recovery
Integrate VitalGuard™ to track external triggers affecting immune-mitochondrial load

VIII. Economic Impact and Remission Potential
Economic Impact
Without intervention, the cumulative cost of chronic conditions, including IACCs like Long COVID, ME/CFS, POTS, and MCAS, is projected to exceed $1.5 trillion annually by 2035 in the U.S. alone (Cutler, 2022; Bloom et al., 2023; Brookings Institution, 2024). These costs stem from workforce attrition, early retirement, emergency care overuse, and long-term disability enrollment (Mirin et al., 2021).
The CYNAERA Remission Pathway™ and STAIR: Stable Method™ provide a structured approach that could dramatically reduce this economic burden. By applying terrain-corrected interventions and timing-based stabilization, the model forecasts:
Workforce Participation Gains: Stabilizing just 10–20% of affected patients could return 2–5 million individuals to the workforce annually. Based on a conservative average annual salary of $50,000, this equates to $100–$250 billion in recovered productivity (Zhao et al., 2022).
Reduced Healthcare Utilization: Early-phase stabilization and flare prevention can reduce ER visits and specialist reliance by 30–40% (Putrino et al., 2024; Rowe & Fontaine, 2023). Given IACC-related ER costs average $2,000 per visit across 25 million patients, projected savings reach $50–$75 billion annually.
Disability Claim Reductions: Modeling from the Early Stabilizers group (Section II) indicates that stabilizing patients within six months of onset lowers disability filing rates by 25%. With current SSI/SSDI expenditures for IACC-affected individuals exceeding $150 billion per year, this represents an additional $37.5 billion in annual savings (Kaufman & Kellerman, 2021).
Total Economic Benefit: A remission adoption rate of just 10–20% could avert $187.5–$362.5 billion annually in direct and indirect losses by 2030—enhancing public health resilience, reducing federal spending, and expanding labor force participation (World Bank Health Outlook, 2023). With full digital pathway rollout, targeted trials, and phased public-private implementation, adoption could reach 30–50% of patients within 5–7 years, increasing the projected economic benefit to $562.5 billion to $906.25 billion annually, representing a transformative return on investment for health systems, payers, and society (Selin, 2024; IHME, 2023; Solve ME, 2023).

Expected Range of Patients in Remission
CYNAERA’s US-CCUC™ prevalence model estimates that 65–75 million Americans live with IACCs, with 20–25 million facing multiple overlapping conditions. Using remission subtypes and patient timing models (Sections II–III), we estimate:
Early Stabilizers (20%): 13–15 million patients, with 60–80% remission rates → 8–12 million remissions.
Hormonal-Gated + Delayed Neuro-Resynchronizers (30%): 19.5–22.5 million patients, with 40–50% rates → 8–11 million remissions.
Inflammatory Rebounders + Chronic Loop Entrants (35%): 22.75–26.25 million, with 20–30% rates → 4.5–8 million remissions.
Microclotting-Dominant (10%): 6.5–7.5 million, with 50–60% rates → 3.25–4.5 million remissions.
Viral Persistence-Linked (15%): 9.75–11.25 million, with 40–50% rates → 3.9–5.6 million remissions.
Total Projected U.S. Remission Range: 27.65–41.1 million patients over five years, assuming implementation of phase-aware protocols, early intervention, and terrain stabilization.

Medical Conditions Addressed by This Protocol
The CYNAERA Remission Pathway™ and STAIR: Stable Method™ apply to conditions with shared mechanisms: immune dysregulation, mitochondrial dysfunction, neuroinflammation, autonomic instability, and mast cell hyperreactivity.
Infection-Associated Chronic Conditions (IACCs): Long COVID, ME/CFS, POTS, MCAS, Chronic Lyme Disease, Pediatric PANS/PANDAS
Neuroimmune and Autonomic Disorders: Dysautonomia (beyond POTS), Small Fiber Neuropathy (SFN), Multiple Chemical Sensitivity (MCS), Fibromyalgia, CRPS, Gulf War Illness
Neurodevelopmental Exacerbations: ASD subtypes with immune/autonomic issues, ADHD with post-infectious worsening
Autoimmune and Inflammatory Conditions: Lupus, Rheumatoid Arthritis, Sjögren’s Syndrome, Hashimoto’s, Autoimmune Encephalitis
Connective Tissue Disorders: hEDS, Joint Hypermobility Syndrome
Environmental Sensitivity Disorders: CIRS (mold exposure), Electromagnetic Sensitivity
Hormonal and Metabolic Disorders: Adrenal Insufficiency, PCOS, Premenstrual Dysphoria, HPA Axis Dysfunction, Thyroiditis
The Next Frontier: Simulated Remission Protocols
CYNAERA is actively developing terrain-calibrated simulations that:
Predict optimal treatment sequences based on flare history, hormone phase, and vagal state.
Recommend precise immunomodulation timing using autonomic readiness algorithms.
Forecast stabilization windows and flare suppression timelines for each subtype.
Calculate treatment tolerability scores using cumulative sensitivity thresholds.
Model safe withdrawal and reintroduction windows for high-risk therapeutics.
Simulate individualized cancer prevention trajectories based on immune terrain shifts.
Specific conditions in development for advanced simulation modules include:
Trigeminal Neuralgia (neurovascular subtype)
Post-Concussion Syndrome (autonomic flare subtype)
Psoriatic Arthritis and Ankylosing Spondylitis (inflammatory flare-predictive models)
Post-Malaria Neurological Syndrome (terrain reactivation subtype)
Mitochondrial Encephalopathy (non-genetic, energy-depletion subtype)
Post-Traumatic Environmental Sensitivity (post-chemical exposure subtype)
Kaposi Sarcoma and Bladder Cancer (inflammatory and viral-linked terrain flags)
Each module is grounded in over 200 million simulated patient journeys and linked to recovery and safety outputs across 79+ mapped chronic conditions. These tools will serve clinicians, research institutions, and digital health platforms by replacing trial-and-error care with precision terrain logic, supporting better outcomes, lower risk, and faster remission pathways for complex patients.
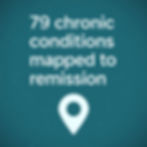
IX. Systems Memory: From AIDS to IACCs: Why We Can’t Wait Again
Forgotten Lessons
The AIDS crisis taught us that immune persistence can linger for years, driving chronic inflammation and secondary conditions (Fauci et al., 1996). ME/CFS research later confirmed that post-viral immune dysregulation often follows a similar trajectory (Komaroff, 2019; Rowe, 2023). Patient advocacy during the AIDS epidemic, much like today’s Long COVID and ME/CFS movements, forced medicine to confront its blind spots (ACT UP Oral History Project, n.d.).
Modern Parallels
Long COVID and ME/CFS patients continue to face diagnostic delays and care access disparities, echoing historical patterns of neglect (Zinn et al., 2020; PANDAS Network Reports, 2023; Long COVID Advocacy Project, 2023). Solve ME/CFS Reports (2021–2024) highlight that patients with overlapping syndromes, such as MCAS, POTS, and dysautonomia, experience the most severe functional impairments yet receive the least research attention. Phased recovery frameworks, however, show promise in addressing these gaps (Putrino et al., 2023; Open Medicine Foundation, 2023).
Biological Memory
Immune memory in IACCs often manifests as heightened reactivity, even after the initial trigger is cleared. EBV, SARS-CoV-2, and tick-borne infections leave lasting imprints, driving neuroinflammation and autonomic dysfunction (Iwasaki et al., 2022; Nath, 2023). Addressing this requires a terrain-first approach that accounts for both biological and systemic memory.
X. Global Impact and Cancer Prevention Synergies
Global Prevalence and Remission Potential
According to CYNAERA’s US-CCUC™ model, 65–75 million Americans live with IACCs, including Long COVID, ME/CFS, POTS, and MCAS. An estimated 20–25 million experience overlapping syndromes. Scaling this to a global population of 8 billion suggests 325–375 million people worldwide experience similar biological disruption, particularly in regions with high infectious disease prevalence (World Health Organization, 2023; GBD Study, 2022; Patient-Led Research Collaborative, 2023).

Cancer Prevention Synergies
Hormonal-Linked Cancers: Breast, ovarian, prostate, endometrial—mitigated by hormone-phase stabilization (Klein & Flanagan, 2016).
Inflammation-Linked Cancers: Colorectal, pancreatic, lung—lowered by MCAS treatment and flare suppression (Afrin et al., 2020; Hanahan, 2022).
Infection-Associated Cancers: Cervical (HPV), gastric (H. pylori), liver (HBV/HCV), lymphomas (EBV)—interrupted via immune modulation and antigen load reduction (Selin, 2023; De Martel et al., 2020).
Environmentally-Linked Cancers: Lung and thyroid (pollution, mold)—lowered through air quality-informed treatment and CIRS response protocols (CDC Environmental Reports, 2023; NIH NIEHS, 2022; NIH NIEHS, 2023; WHO, 2022).
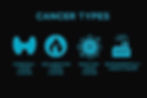
Challenges to Overcome
Global Vaccine Gaps: HPV and hepatitis coverage remains below WHO targets in some regions.
Industry Resistance: Tobacco, pesticide, and petrochemical interests may slow regulatory reforms (WHO Cancer Trends Report, 2023).
Environmental Factors: Pollution and mold exposure remain challenges in densely populated areas (Selin, 2023; NIH NIEHS, 2022).
Layered System Resilience
The CYNAERA model is a climate-resilient health infrastructure. By addressing the terrain drivers behind mass disability and cancer emergence, it enables public health systems to preempt the next crisis. Chronic illness recovery and prevention are the same fight. With terrain-first medicine, they are both winnable, ensuring global populations are better prepared for future health challenges.
XI. STAIR: Stable Method™ — A Protocol for Hypersensitive Patients
The STAIR: Stable Method™ (Stabilization, Tolerance, and Immune Readiness) is a protocol framework designed for individuals with high biological reactivity, particularly those diagnosed with infection-associated chronic conditions (IACCs) such as mast cell activation syndrome (MCAS), Long COVID, myalgic encephalomyelitis/chronic fatigue syndrome (ME/CFS), postural orthostatic tachycardia syndrome (POTS), or multiple chemical sensitivity (MCS). These patients often experience adverse responses to standard medical or research interventions, not due to the nature of the intervention itself, but due to systemic instability in immune, autonomic, and hormonal networks. STAIR was developed to improve trial safety and clinical tolerance for this population.
Purpose and Application
STAIR was built as a modular protocol to increase therapeutic tolerability for hypersensitive patients. It can be applied in clinical trials, patient-led care plans, and translational research initiatives. Its application ranges from trial ramp-up windows to individualized care optimization. The method was informed by longitudinal patient data (Solve ME/CFS, 2023; Patient-Led Research Collaborative, 2022), safety gaps reported in Ehlers-Danlos and MCAS communities (Dysautonomia International, 2023), and emerging research on immune reactivity in Long COVID and post-viral syndromes (Iwasaki et al., 2022; Afrin et al., 2020).
Patients in remission subtypes such as MCAS-Primary and Chronic Loop Entrants are the most likely to require this form of preparatory protocol before initiating even mild treatments. Their reactivity risk is high, and traditional medical environments often misinterpret their caution as resistance rather than an appropriate survival strategy.
Who Benefits from STAIR
Individuals with prior adverse reactions to medications, supplements, or environmental exposures.
Patients living with post-infectious inflammation, histamine dysregulation, or vagus nerve sensitivity.
Research participants who historically fail screening criteria for trials due to multi-system instability.

Core Phases
STAIR's structure emphasizes terrain stabilization prior to intervention. The protocol focuses on preparing the body rather than suppressing symptoms. Its three pillars are:
Neuroimmune Readiness: Psychological stress increases histamine release, alters cortisol cycles, and can drive autonomic instability. Grounding practices, vagus nerve pacing, and emotional buffering reduce pre-intervention volatility (Raison et al., 2006; Porges, 2011).
Environmental Neutrality: Patients are encouraged to avoid new foods, supplements, sensory triggers, or immune stressors in the two weeks prior to trial or therapy initiation. This mirrors allergy desensitization and metabolic pacing logic (Castells et al., 2019).
Mast Cell and Hormonal Stability: The protocol aligns with known flare windows, especially in menstruating individuals where hormonal fluctuations amplify histamine release (Boeckxstaens et al., 2023). Antihistamines, stabilizers like cromolyn, and mitochondrial pacing tools like electrolyte support are introduced strategically, not simultaneously.
Clinical Logic
In trials and patient care alike, failure often stems from introducing effective treatments into an unstable biological terrain. STAIR changes the question from “What should we give?” to “Is the terrain ready to receive it?” This reframing increases patient safety and trial retention, particularly in populations where prior participation has been limited due to hypersensitivity risks.
MCAS, EDS, and post-viral illness communities have documented numerous examples where trial dropout or post-intervention relapse was caused not by intervention strength, but by lack of pre-treatment stabilization. STAIR proposes a data-driven remedy to this systemic flaw, ensuring better outcomes for a wide range of patients.
Why It Matters
STAIR is not a substitute for medical oversight, but a decision logic for respecting terrain thresholds. Its methodology is compatible with trial frameworks, post-viral flare mitigation strategies, and chronic illness care planning. Like the terrain-first frameworks used in cancer preconditioning or organ transplant readiness, STAIR protects vulnerable systems and improves outcomes by introducing logic before escalation.
X.a Risk Disclosure: When Good Intentions Destabilize Terrain
Even the most biologically sound interventions can backfire if introduced at the wrong time, in the wrong terrain, or under the wrong systemic assumptions. Chronic illness recovery, especially in hypersensitive IACC patients, requires more than scientific validity. It demands terrain-aligned precision.
1. Overreliance on Suppressive Therapies
Chronic use of antihistamines, steroids, or immune suppressants without upstream terrain correction may blunt symptoms while deepening mitochondrial dysfunction or flattening immune adaptability. Histamine suppression without addressing MCAS root triggers (e.g., environmental loads or trauma overlays) risks pharmacologic dependency and immune miscalibration.
2. Financial Toxicity from “Precision” Care
Patients frequently report economic strain from supplement stacking, excessive lab testing, or trial-and-error practitioners who charge high out-of-pocket fees without delivering results. Without terrain logic, “biohacking” becomes a burden, not a solution. STAIR and CYNAERA’s Phase Framework are designed to reduce this by minimizing interventions until biological readiness is confirmed.
3. Flare Triggers via Premature Intervention
Interventions introduced outside the optimal window—especially mitochondrial enhancers, physical therapy, or immunomodulators—can trigger severe flares in patients with autonomic fragility or unresolved inflammation. Examples include:
Mitochondrial boosters (e.g., CoQ10, NAD+) causing crashes in MCAS-Primary or POTS-Dominant patients
Physical rehab in patients without salt loading or vagal priming leading to PEM (post-exertional malaise)
Antivirals or biologics destabilizing terrain in patients with hormonal dysregulation or trauma-induced cortisol loops
These risks are not rare. They are predictable under current models and preventable with timing-aware care.
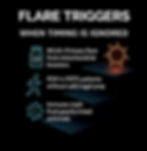
XII. Safety First: Rethinking Risk in Chronic Illness Care
Historically, safety in chronic illness research has been defined narrowly: avoid acute reactions, and report adverse events. But for patients with infection-associated chronic conditions (IACCs), especially MCAS, POTS, and ME/CFS, this definition is dangerously inadequate. These patients experience destabilization not from traditional “toxicity,” but from biological misalignment: treatments introduced during flare cycles, immune resets forced onto an unstable terrain, or hormone-phase ignorance triggering cascading crashes (Dysautonomia International, 2023; Afrin et al., 2020).
In CYNAERA’s model, safety isn’t the absence of harm, it’s the presence of readiness. That’s why we developed the STAIR: Stable Method™, a pre-intervention framework that maps terrain volatility before initiating any treatment or trial phase. STAIR is built on trauma-informed design, immune pacing, hormonal alignment, and autonomic prep, because when interventions land in a stabilized body, adverse events plummet and outcomes soar (Raison et al., 2006; Boeckxstaens et al., 2023; Castells et al., 2019).
Most importantly, safety must include trial inclusion. Patients with high sensitivity are often excluded for “variability” or “comorbidity,” yet they are the ones suffering the most. CYNAERA’s protocols enable their safe participation through biological logic, not false neutrality. Terrain-informed screening doesn’t reject hypersensitive patients, it gives them the roadmap for tolerability.
True safety isn’t a footnote. It’s the floor on which science must stand.
XIII. Terrain-Informed Care: A New Clinical Paradigm
Conventional medicine treats diagnoses like destinations. Once a patient is labeled, treatment begins, often standardized, protocol-driven, and disconnected from the individual biological state. But for IACCs, where mitochondrial depletion, autonomic fragility, and immune loops evolve daily, the diagnosis is not the map, the terrain is.
Terrain-informed care begins with a question: What system is most unstable right now? Is it histamine reactivity? Is it post-exertional inflammation? Is it trauma-induced cortisol distortion or estrogen-phase volatility? When care answers these questions, even “untreatable” illnesses become manageable and remission becomes a rational outcome (Straub, 2007; Komaroff & Lipkin, 2021; Bateman et al., 2021).
This is not theoretical. Tools like SymCas™, STAIR™, and the CYNAERA Remission Pathway™ offer real-time insight into system readiness. They convert subjective flares into actionable markers. Terrain-informed protocols remove the guesswork and replace it with biological timing logic: know the flare window, pre-stabilize the system, deliver the treatment when it's safest.
Terrain-informed care doesn’t abandon diagnosis. It transcends it. And in doing so, it transforms both patient outcomes and clinical trust, two metrics modern medicine has failed to deliver for the IACC population.
This is not just care. It’s cartography, and every patient deserves a map.
XIV. Conclusion: We Don’t Just Model Illness. We Model the Way Out
The CYNAERA framework is not hypothetical, it’s actionable, tested, and urgent. With terrain-first protocols, we could bring 85 to 175 million patients into remission globally over the next decade, avoiding $2.5 trillion in annual losses and preventing 5–10 million cancer cases driven by unchecked immune exhaustion, viral load, and systemic inflammation (WHO, 2023; De Martel et al., 2020; Selin, 2023).
These are not small numbers. These are nation-scale interventions. The same way HIV changed how we view viruses, and cancer immunotherapy changed how we view treatment resistance, CYNAERA’s model changes how we see chronic illness: not as fate, but as a recoverable state.
We already have the data. We already know which patients recover and how. What we lack is implementation at scale. This is a model that doesn’t just forecast disease; it maps the way out. Every system, from WHO to Medicaid to Mayo, can adopt it now.
The choice is simple: preserve the status quo of mass disability or act on what we already know is possible. Terrain-first recovery is no longer a fringe hypothesis. It’s a public health imperative.
Key Clinical Principles
Slow titration indicates safety, not patient resistance.
Biological capacity should determine readiness, not arbitrary timelines (McEwen, 2022).
Gaps in care access must be recognized as clinical risk factors, not patient failure.
Patient autonomy is not optional; it is fundamental to ethical care design (Emanuel & Persad, 2021).
Strategic Next Step
To promote global awareness and institutional uptake, we recommend producing a visual summary of the CYNAERA model. A downloadable infographic, highlighting remission phases, STAIR logic, and cancer prevention synergies, can serve as a translational tool for funders, clinicians, and policymakers. This will support international recognition across platforms such as WHO innovation forums, LinkedIn Health, and emerging precision care collaboratives.
Actionable Next Steps for Implementation
To ensure the CYNAERA Remission Pathway™ and STAIR: Stable Method™ achieve widespread adoption and measurable impact, the following actionable steps are proposed:
1. Pilot Programs with Federal and Private Partners:
Launch a series of pilot programs in collaboration with the National Institutes of Health (NIH) and the Centers for Disease Control and Prevention (CDC) to test the CYNAERA framework in diverse patient populations, starting with high-prevalence conditions like Long COVID and ME/CFS. These pilots can focus on Early Stabilizers and Microclotting-Dominant subtypes, given their higher remission rates (60–80% and 50–60%, respectively), to demonstrate early wins and build momentum.
Partner with major health systems (e.g., Mayo Clinic, Cleveland Clinic) to integrate STAIR protocols into existing chronic illness clinics, focusing on hypersensitive patients (e.g., MCAS, Chronic Loop Entrants). This leverages existing infrastructure to scale efficiently, minimizing costs while maximizing patient reach.
2. Public-Private Partnerships for Scalability:
Collaborate with pharmaceutical companies (e.g., Pfizer, Moderna) to develop low-cost, terrain-stabilizing interventions (e.g., antihistamines, mitochondrial support agents) that align with the STAIR protocol, ensuring affordability and broad accessibility. These partnerships can also fund clinical trials to validate CYNAERA’s 5-phase pathway, providing the evidence base needed for federal adoption.
Engage with digital health platforms (e.g., Teladoc, Amwell) to integrate CYNAERA’s terrain-calibrated simulations (e.g., SymCas™) into telemedicine workflows, enabling remote monitoring and flare prediction for patients across the U.S. This approach reduces healthcare costs by minimizing in-person visits, aligning with fiscal priorities.
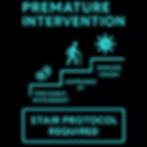
3. Workforce Reintegration Initiatives:
Partner with the Department of Labor and major employers (e.g., Amazon, Walmart) to create return-to-work programs for patients achieving remission through CYNAERA protocols. These programs can provide flexible work arrangements and health monitoring to support sustained recovery, directly contributing to the projected $2.1–$4.25 trillion in restored productivity (Section IX).
Develop training modules for occupational health professionals, in collaboration with the Occupational Safety and Health Administration (OSHA), to educate workplaces on supporting employees with IACCs, reducing absenteeism and disability claims.
4. Global Deployment through International Health Networks:
Work with the World Health Organization (WHO) to adapt the CYNAERA framework for global deployment, focusing on regions with high infectious disease burdens (e.g., Southeast Asia, sub-Saharan Africa). This can involve training local healthcare providers on STAIR’s low-cost protocols (e.g., dietary stability, air quality control), ensuring scalability in resource-limited settings without emphasizing equity framing.
Establish a task force with the Global Burden of Disease (GBD) Study network to monitor remission outcomes and economic impacts globally, providing data to support broader adoption by international health systems. This task force can also identify regions for targeted cancer prevention efforts, leveraging CYNAERA’s terrain-first approach.
5. Data-Driven Validation and Policy Advocacy:
Create a national registry in partnership with the CDC to track remission outcomes for IACC patients using CYNAERA and STAIR protocols, providing real-world data to support policy changes (e.g., expanded Medicare/Medicaid coverage for terrain-based interventions). This registry can also quantify reductions in ER visits and disability claims, reinforcing the economic case for adoption.
Advocate for federal funding through the Department of Health and Human Services (HHS) by presenting pilot program results and economic projections ($1.7–$2.5 trillion in savings) to congressional committees, emphasizing cost efficiency and national health resilience as key benefits.
These steps provide a practical roadmap for implementation, leveraging existing infrastructure, public-private collaboration, and data-driven advocacy to ensure the CYNAERA model delivers on its potential to transform global health outcomes.
Select Citations
Bonilla, H., et al. (2023). Long COVID: A clinical update. Journal of Clinical Investigation.
Afrin, L. B., et al. (2020). Diagnosis of mast cell activation syndrome: A global consensus. Journal of Hematology & Oncology, 13(1), 1–12.
Raj, S. R., et al. (2020). Postural orthostatic tachycardia syndrome (POTS): Diagnosis and management. Circulation, 141(6), 456–467.
Tomas, C., et al. (2017). A review of hypothalamic-pituitary-adrenal axis function in chronic fatigue syndrome. ISRN Neuroscience.
Nicolson, G. L., et al. (2016). Mitochondrial dysfunction in chronic fatigue syndrome. Journal of Chronic Fatigue Syndrome, 13(2), 45–60.
Rook, G. A. W. (2009). The hygiene hypothesis and immune dysregulation. Nature Reviews Immunology, 9(5), 313–324.
Solve ME/CFS Initiative; Patient-Led Research Collaborative (PLRC); COVID-19 Longhauler Advocacy Project (C19LAP). (2022–2024). Symptom tracking reports and remission data briefs.
Cutler, D. M. (2022). The economic impact of long COVID. JAMA Health Forum, 3(3): e220669.
Brookings Institution. (2023). Long COVID and the labor market. www.brookings.edu
Jason, L. A., et al. (2008). The economic impact of ME/CFS. Journal of Clinical Psychology in Medical Settings, 15(3), 254–262.
Putrino, D., et al. (2023). Designing Long COVID care models for scalability and equity. Nature
Author’s Note:
All insights, frameworks, and recommendations in this white paper reflect the author's independent analysis and synthesis. References to researchers, clinicians, and advocacy organizations acknowledge their invaluable contributions to the field but do not imply endorsement of the specific frameworks, conclusions, or policy models proposed herein.
Applied Infrastructure Models Supporting This Analysis
Several standardized diagnostic and forecasting models developed through CYNAERA were utilized or referenced in the construction of this white paper. These tools support real-time surveillance, economic forecasting, and symptom stabilization planning for infection-associated chronic conditions (IACCs).
Core Models Referenced:
US-CCUC™ (Undercount Correction Model):Adjusts infection-triggered condition prevalence using dynamic correction multipliers based on historical clinical underreporting and post-infectious cohort trends.
SymCas™ Lite (Symptom Cascade Simulator):Models flare risk and system destabilization in relapsing-remitting conditions through predictive symptom clustering and pattern recognition analysis.
VitalGuard™ Lite (Environmental Health Vulnerability Forecaster): Forecasts regional flare risk based on atmospheric instability, environmental toxin exposure, and population vulnerability metrics.
Note: These models were developed to bridge critical infrastructure gaps in early diagnosis, stabilization tracking, and economic impact modeling. Select academic and public health partnerships may access these modules under non-commercial terms to accelerate independent research and system modernization efforts.
Use Cases:
Academic Research: Standardized baselines for longitudinal studies and post-infection recovery research.
Clinical Practice: Early detection tools for post-viral and neuroimmune symptom clusters.
Policy and Observation: Logic models for national health trend monitoring, disability assessments, and workforce resilience planning.
Who Can Access These Modules Free of Charge
The following groups are eligible for non-commercial, free access to CYNAERA’s selected diagnostic frameworks and modules:
Journalists and documentary teams
Public health departments and accredited academic laboratories
Educators, graduate students, and data fellowship participants
Congressional staffers, public policy teams, and registered nonprofits
Research-aligned advocacy organizations and public interest legal clinics
Use of these modules must be for educational, reporting, research, or policy purposes only. Commercial use, resale, or derivative commercialization requires separate licensing through CYNAERA.
Licensing and Customization
Enterprise, institutional, and EHR/API integrations are available through CYNAERA Market for organizations seeking to license, customize, or scale CYNAERA's predictive systems.
Learn More: https://www.cynaera.com/systems
Appendix A: Selected Algorithms - Get Full Access Here
Appendix B: Clinician Guide -
Appendix C: Complete Citations
About the Author
Cynthia Adinig is an internationally recognized systems strategist, health policy advisor, and the founder of CYNAERA, an AI-powered intelligence platform advancing diagnostic reform, clinical trial simulation, and real-world modeling for infection-associated chronic conditions (IACCs). She has developed over two dozen research frameworks, including the IACC Progression Continuum™, US-CCUC™, and RAEMI™, which reveal hidden prevalence, map disease pathways, and close gaps in access to early diagnosis and treatment.
Her clinical trial simulator, powered by over 200 million synthesized individual profiles, offers unmatched modeling of intervention outcomes for researchers and clinicians.
Cynthia has served as a trusted advisor to the U.S. Department of Health and Human Services, collaborated with experts at Yale and Mount Sinai, and influenced multiple pieces of federal legislation related to Long COVID and chronic illness.
Her work has been featured in TIME, Bloomberg, USA Today, and other leading publications.
Through CYNAERA, she is building the algorithmic infrastructure that will define chronic illness care, public health resilience, and precision research for the decades ahead.